
An international team of engineers has developed an innovative, scalable method for creating topography-patterned aluminum surfaces, enhancing liquid transport properties critical for applications in electronics cooling, self-cleaning technologies and anti-icing systems. The research, published recently in Langmuir and conducted by groups at Rice University and the University of Edinburgh as part of the Rice-Edinburgh Strategic Collaboration Awards program, demonstrates how cost-effective vinyl masking techniques can produce surfaces with high-resolution wettability contrast, paving the way for improved phase-change heat transfer applications.
The research team developed a novel technique using blade-cut vinyl masking and commercially available lacquer resin combined with scalable physical and chemical surface treatments to create patterned aluminum surfaces. These surfaces exhibit distinct wettability contrasts, significantly improving the droplet shedding during condensation. The patterns, with feature sizes as small as 1.5 mm, offer a range of wettability behaviors — from superhydrophobic to hydrophilic — depending on the treatment.

“This method represents an important step in tailored surface engineering,” said Daniel J. Preston, assistant professor of mechanical engineering at Rice and a co-corresponding author of the paper along with Geoff Wehmeyer, assistant professor of mechanical engineering at Rice, and Daniel Orejon from the University of Edinburgh. “By enabling precise control over surface wettability and thermal properties, we are opening new doors for scalable manufacturing of advanced heat transfer surfaces.”
The research employed a multistep methodology to develop and analyze the patterned aluminum surfaces. Vinyl masks were first applied to polished aluminum substrates, followed by a two-step etching process that created micro- and nanotextured zones. The team then used advanced imaging techniques to characterize the patterns’ resolution and wettability properties. To evaluate performance, condensation visualization experiments demonstrated enhanced droplet shedding on the patterned surfaces compared to homogeneous ones. Additionally, thermal emissivity mapping using infrared thermography revealed significant contrasts in emissivity between smooth and textured regions, highlighting the surfaces’ potential for advanced thermal management applications.
“Aluminum is widely used in thermal management devices like heat exchangers due to its high conductivity, low density and low cost,” said Wehmeyer. “Our method adds a new dimension to its functionality by integrating surface patterning that is both cost-effective and scalable, allowing engineers to fine-tune the condensation heat transfer. This work brought together expertise from Edinburgh and Rice to develop and characterize these advanced surfaces.”
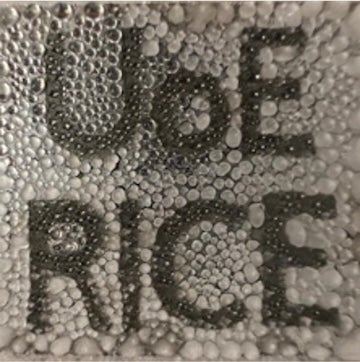
The findings have significant implications for industries that depend on phase-change heat transfer with applications in everyday technologies. In electronics cooling, enhanced droplet shedding reduces thermal resistances associated with large droplets during condensation, which could enable new cooling strategies for data center servers or other electronic devices that rely on effective heat dissipation to prevent overheating. Tailored thermal emissivity patterns optimize heat dissipation in high-temperature environments, benefiting systems such as automotive engines and aerospace components. Additionally, superhydrophobic regions expedite water removal, preventing ice formation on critical surfaces like airplane wings, wind turbines and power lines during freezing conditions. These advancements offer practical solutions to enhance the performance and reliability of technologies people use and depend on every day.
“Traditional methods like photolithography are typically expensive and limited to small areas,” Preston said. “Our technique uses affordable, accessible materials to create intricate patterns on larger surfaces, making it suitable for industrial applications and a promising technique for designing next-generation condensers and heat exchangers.”
The lead authors on the work are Trevor Shimokusu (Rice mechanical engineering doctoral graduate, now a faculty member at the University of Hawaii) and Hemish Thakkar (Rice graduate with a double major in chemistry and mechanical engineering, now a doctoral student at Princeton University).
This work was supported by the Rice-Edinburgh Strategic Collaboration Award program, a NASA Space Technology Graduate Research Opportunities award and grants from the National Science Foundation.